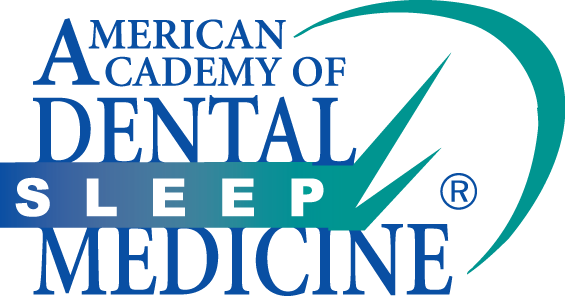
Review Article, Issue 3.1
Pathogenesis of Upper Airway Obstruction and Mechanical Intervention during Sedation and Sleep
http://dx.doi.org/10.15331/jdsm.5364
Takao Ayuse, DDS, PhD1 ; Jason Kirkness, PhD2 ; Takuro Sanuki, DDS, PhD1 ; Shinji Kurata, DDS, PhD1 ; Ichiro Okayasu, DDS, PhD1
Faculty of Dentistry, University of Montreal, Quebec, Canada
ABSTRACT
Upper airway patency is balanced by both oro-pharyngeal muscle activity and the intraluminal negative pressure caused by respiratory muscles during sleep and anesthesia. The mechanical upper airway properties may become the dominant factor governing upper airway collapsibility both during sleep and sedation due to the significant depression of consciousness and the impairment of neural mechanisms controlling compensatory neuromuscular responses. It is recognized that the pathogenesis of upper airway obstruction, due to alteration of consciousness during sleep and sedation, might be similar. Furthermore, the clinicians who manage obstructive sleep apnea patients should also be aware of the pathogenesis of upper airway obstruction during sleep. Anesthesiologists and surgeons who are responsible for airway management during procedures under sedation and the perioperative period should therefore be well versed with the physiological and pathophysiological mechanisms affecting upper airway patency. This review article presents the current understanding of mechanisms for maintaining upper airway patency during sleep and sedation based on the similarity of the pathophysiology governing upper airway patency. Possible mechanical interventions based on a quantitative analysis of upper airway collapsibility analyzing inspiratory flow limitation are also discussed.
Keywords:
upper airway obstruction, sleep, sedation, obstructive sleep apnea, maxillofacial surgery
Citation:
Ayuse T, Kirkness J, Sanuki T, Kurata S, Okayasu I. Pathogenesis of upper airway obstruction and mechanical intervention during sedation and sleep. Journal of Dental Sleep Medicine 2016;3(1):11–19.
INTRODUCTION
Upper airway patency depends on an appropriate balance between the dilating force of the pharyngeal muscles and the collapsing force of negative intraluminal pressure, which is generated by respiratory pump muscles. It is well accepted that maintenance of upper airway patency is a critical issue during sleep and sedation, because loss of consciousness may induce a depression of central respiratory output1–3 by altering hypercapnic and hypoxic ventilatory drives, and it may decrease muscle contractility via cellular mechanisms by blocking sarcolemmal sodium channels.4 Furthermore, it has been suggested that the neuromuscular activity of upper airway dilator muscles, such as the genioglossus and geniohyoid, may be affected by depression of hypoglossal motor neurons, which regulate tonic activation of these muscles. These influences, in association with depression of consciousness during sleep and sedation, may result in hypopnea and apnea due to upper airway obstruction. It has been suggested that the pathophysiology of upper airway obstruction might be similar in sleep and sedation.5,6 Furthermore, the maintenance of mechanical upper airway properties may contribute significantly to upper airway patency. Therefore, understanding of the pathogenesis of upper airway obstruction may help establish clinical diagnostic and treatment methods in both sleep and sedation. Clinicians who are responsible for airway management during sleep and sedation should, therefore, be familiar with the physiological mechanisms influencing upper airway patency. This review article presents the current understanding of mechanisms for maintaining upper airway patency and discusses the developmental aspects of the mechanisms, based on a quantitative analysis of upper airway collapsibility using the concept of flow limitation. Furthermore, the similarity of the pathophysiology of upper airway obstruction between sleep and anesthesia based on the features of upper airway obstruction is also discussed. Lastly, the implications of the changes of upper airway patency by mechanical interventions during sleep and anesthesia are discussed.
PATHOGENESIS OF UPPER AIRWAY OBSTRUCTION UNDER ANESTHESIA
The anatomical structure of the upper airway is characterized by a balanced combination of soft tissue components (tongue, soft palate, and pharyngeal mucosa) and bony structure components (maxilla, mandible, and vertebrae).7 Upper airway patency is determined by precise interaction between the mechanical properties of the upper airway (anatomical mechanisms) and neural regulation of pharyngeal dilator muscle activity (neural mechanisms). Previously, the Neural Balance Model8 and the Anatomical Balance Model9 were introduced to understand how the upper airway is protected against upper airway obstruction during anesthesia.RESPONSE TO ACUTE AND SUSTAINED PARTIAL UPPER AIRWAY OBSTRUCTION
Upper airway obstruction during sleep plays a pivotal role in the pathogenesis of obstructive sleep apnea10 and is caused by structural defects and disturbances in neuromuscular control.6 Upper airway obstruction can elicit neuromuscular responses that mitigate and/or compensate for the obstruction. Under conditions of upper airway obstruction (inspiratory airflow limitation), immediate responses in respiratory timing indices can help restore ventilation11 and blunt disturbances in gas exchange.12 Nevertheless, the impact of respiratory pattern responses on ventilation during periods of upper airway obstruction remains unclear. It has recently been suggested that the respiratory cycle, but not the respiratory rate, determines the individual’s ability to compensate for inspiratory airflow limitation during sleep13 and during propofol anesthesia,5 and it may represent a quantitative phenotype for obstructive sleep apnea susceptibility.It has also been indicated that the compensatory neuromuscular response to upper airway obstruction is partly intact during propofol anesthesia with spontaneous breathing.5 Interestingly, it has been reported that there was a significant difference in the compensatory neuromuscular response to upper airway obstruction between male and female subjects during midazolam sedation.14 In natural NREM sleep, there was a significant difference in the compensatory neuromuscular response to upper airway obstruction between OSA patients and healthy subjects.6 It is easy to expect that patients with depressed neuromuscular activity, such as cerebral palsy patients, may have weaker effects of this function. Furthermore, similar to OSA, aging may affect the magnitude of the compensatory neuromuscular response to upper airway obstruction.
During natural sleep, when sustained partial obstruction or complete obstruction occurs, the obstructed upper airway is re-opened by a brief arousal response, resulting in the return of muscle tone.15 During sedation, the decrease in muscle tone associated with reduction of consciousness is compounded by specific drug-induced inhibition of upper airway neural and muscular activity and suppression of protective arousal responses. This depression of the arousal reflex during sedation even more than during NREM sleep might increase upper airway obstruction, such that external mechanical intervention may be needed to overcome the obstruction. Furthermore, the role of chemoreceptors from carotid body and retroambiguus nucleus may be depressed during sedation and anesthesia.16 Although the arousal response against sustained upper airway obstruction is a fundamental defensive mechanism in the compensatory neural system to maintain upper airway patency, this arousal response may be even more depressed as anesthetic depth increases.
EVALUATION OF UPPER AIRWAY COLLAPSIBILITY
Concept of Flow Limitation and Critical Closing Pressure (PCRIT) (Figures 1–4)
Upper airway collapsibility is evaluated by static imaging analysis via 2 dimension computerized tomography (CT) or magnetic resonance imaging (MRI), and 2 dimension cephalography X-ray, as well as dynamic analysis using the pressureflow/volume relationship and pressure-cross-sectional area curves.
Among these quantitative analyses of upper airway patency, determination of the airway pressure that causes airway collapse and inspiratory airflow limitation in patients with obstructive sleep apnea has been used extensively in sleep apnea research. The application of negative airway pressure to determine the collapsibility of the upper airway using pressure-flow relationships, as seen with flow limitation or complete obstruction, has been used during anesthesia and sleep. The concept of critical closing pressure (PCRIT) arises from modeling the upper airway as a simple collapsible tube and generation of multipoint pressure flow (P-Q) relationships, which are then used to assess upper airway patency.17 Schwartz et al.18 indicated that the upper airway patency can be explained by a Starling resistor model (Figure 1), in which inspiratory flow limitation occurs once upper airway upstream pressure falls below a critical closing pressure (PCRIT). It has been shown that PCRIT (representing nasal pressure at zero flow, an index of upper airway collapsibility) and resistance (which reflects the degree of upper airway narrowing upstream to the site of collapse) are key elements governing upper airway patency. PCRIT can be estimated for the quantitative evaluation of upper airway patency based on nasal pressure and maximum inspiratory airflow in laboratory and has been validated for clinical usage or a research tool, even though this is not easy to extract from ordinary respiratory parameters. It is worth mentioning the clinical significance of PCRIT. In fact, a ~5-cm H2O decrease in PCRIT, due to increased neuromuscular activity, has the same stabilizing effect as applying ~5 cm H2O of continuous positive airway pressure (CPAP) in reversing upper airway obstruction in obstructive apnea patients. It was previously suggested that a change in PCRIT of ~5 cm H2O due to neuromuscular activity is clinically relevant,6 since this represents the magnitude of the response required to convert either obstructive apneic events to less severe hypopneic events or hypopneic events to stable breathing. Because the PCRIT measurements can be clinically relevant for evaluating upper airway collapsibility in patients during anesthesia and sleep, this method might be useful for investigating the pathophysiology of upper airway obstruction occurring during monitored anesthesia care. The advantage of this model is that it gives a global measure of upper airway collapsibility that includes both the structural and neuromuscular factors that determine upper airway collapsibility. More recently, advanced methods for evaluating both the mechanical properties and the compensatory neuromuscular responses to upper airway obstruction were predicted.5,6 The most recent paper revealed that the PCRIT can be assessed by the analysis software (PCRIT Analysis Software in a numerical computing enviroment with 4th generation programming language: PAS) to streamline PCRIT analysis using quantitative airflow measurement data in clinical cohorts study.19
Figure 1Mechanical analogue of upper airway consists of a two tube with a collapsible segment, upstream (nasal) and downstream (hypopharyngeal) segments. |
Figure 2Diagram of the experimental setup. |
Figure 3A schematic of the experimental protocol for producing upper airflow obstruction. |
Figure 4The change of compensatory neuromuscular response during propofol anesthesia. |
PATIENT FACTORS PREDISPOSING TO UPPER AIRWAY OBSTRUCTION
Patient Position during the Procedure
Supine Position
The supine posture predisposes to upper airway obstruction, as the effects of gravity increase the extra-luminal compressive forces exerted by the tongue, soft palate, and related structures, resulting in narrowing of the retropalatal and retrolingual spaces.20
Head Down Posture:
The table tilt with head down position is recognized as being unfavorable, because of loss of longitudinal tension on the upper airway and fluid displacement into the upper airway region. Fluid displacement from the lower body to upper airway regions may increase upper airway collapsibility. Shepard et al. suggested that fluid accumulation in soft tissues surrounding the upper airway may increase pharyngeal collapsibility in patients with OSA.21 It has also been shown that ~375 mL of fluid displacement from the legs by lower body positive pressure increases upper airway collapsibility by about 7.6 ± 1.9 cm H2O in healthy, non-obese men while awake.22 More recent studies have shown that fluctuation of estrogen and progesterone levels is coupled to fluid shifts from the vascular into the interstitial fluid compartments, causing edema.23–25Neck Flexion
Neck flexion reportedly decreases pharyngeal size and increases passive PCRIT in anesthetized patients.9,26 Walsh et al.26 reported that neck flexion with 10-degree deviation from the neutral position produced a 4.9 ± 3.1 cm H2O increase in passive PCRIT. Head elevation with a pillow seems to dose-dependently improve pharyngeal patency, although the possibility of simultaneous neck flexion would attenuate the beneficial effects of head elevation.27 Accidental neck flexion may easily occur during surgical procedures in the oro-pharyngeal region.Bite (Mouth) Opening
It is essential to keep the mouth open during oral-maxillofacial surgical procedures and dental treatment. However, as has been previously reported, this may cause obstruction.28,29 Mouth opening decreases the space enclosed by the maxilla, mandible, and cervical vertebrae and increases the soft tissue volume inside the bony box, similar to that with neck flexion, since the mandibular movement with mouth opening is essentially the same as that which occurs with neck flexion.9,28 Accordingly, the resultant increase in passive PCRIT is predictable by the possible anatomical imbalance during mouth opening. In fact, passive PCRIT increased significantly by 5.1 cm H2O with the mouth open.28 Mouth opening may change the vector force direction of the pharyngeal dilator muscles. Obviously there are high risks of increased upper airway collapsibility by existence of large tonsils30–32 and macroglossia.33,34Mechanical Displacement of the Tongue
Clinically, the tongue is frequently manipulated during surgical procedures in the mouth, with compression by instruments (tongue retractor) to avoid accidental injury by surgical instruments or to maintain the surgical view. In contrast to the beneficial effect of tongue protrusion on upper airway collapsibility35 during sleep, downward displacement (retrograde) of the tongue in supine position might increase upper airway collapsibility during sleep.36,37 Although the effect of tongue displacement on upper airway collapsibility during anesthesia has not been well established, we should be aware of the potential risk of surgical procedures in the oro-pharyngeal region.Patients’ Individual Anatomical Factors
It is well recognized that obesity, micrognathia, macroglossia and maxillary hypoplasia, acromegaly, Down syndrome, Pierre-Robin syndrome, or other craniofacial abnormalities are major anatomical risk factors for upper airway obstruction. The degree of obstruction depends on the anatomical abnormalities in the pharynx. Furthermore, sex and age may other factors for controlling upper airway patency.Recently, we demonstrated that female patients in the luteal phase of their menstrual cycle had an increased passive PCRIT during propofol anesthesia. This conceivably reflects the development of pharyngeal edema due to the effect of sex hormones.38 Based on evidence that there is a significant increase in edema formation in the upper airway region during the late-luteal phase in premenstrual dysphoric disorders,39 we speculated that upper airway collapsibility may be significantly increased by edema formation40 in the premenstrual phase, especially when there is a reduction of neuromuscular activity during shifts in progesterone level. We believe that our findings may provide new insight into the management of sedation in pregnant women, since they have much higher estrogen levels and significant upper airway edema.
Several studies have shown that surface tension41–43 and saliva production44 are important factors controlling upper airway patency, because surface tension is important for re-opening closed upper airways. These studies demonstrated that reduction of the surface tension in the upper airway mucosa by one-third can reduce the difference between the opening pressure and the closing pressures of the upper airway by 2 cm H2O. Furthermore, our recent study found that an increase of 100-nM phosphatidylcholine decreases surface tension of saliva by ~17 mN/m. Surface tension may be increased in Sjögren syndrome.45 Kirkness et al.42 revealed that changes in surface tension significantly reflect the changes in upper airway opening pressure without affecting the upper airway closing pressure in humans. Deformation of the upper airway by negative transmural pressure during inspiration alters the activity of upper airway mechanoreceptors, causing a reflex increase in upper airway muscle activity. There may be significant influence of inflammation of pharyngeal tissue and neuropathic changes in tissue on reduction of reactivity in maintaining upper airway patency.
EFFECT OF SEDATION ON UPPER AIRWAY COLLAPSIBILITY (PCRIT)
It has been reported that the upper airway tends to get obstructed during sedation. Changes in upper airway patency during sedation appear to vary with the agents used, which include intravenous anesthetics (propofol) and sedative drugs (midazolam).46,47 Midazolam and propofol are common anesthetic agents administered to provide anxiolysis, sedation, and amnesia during interventional procedures due to their rapid onset and limited duration of action. Although the effects of midazolam and propofol anesthesia are believed to be equivalent in terms of upper airway patency47 during moderate levels of monitored anesthesia care, upper airway collapsibility dose-dependently increases as depth of anesthesia increases with each anesthetic agent.46,48 Norton et al.47 suggested that midazolam and propofol anesthesia have the same propensity for causing upper airway obstruction with mild to moderate sedation, based on an analysis using dynamic negative airway pressures. Using PCRIT analysis (Figure 1), we confirmed that upper airway mechanical properties are similar with midazolam (mean value of passive PCRIT = −5.1 cm H2O) and propofol (mean value of passive PCRIT = −4.4 cm H2O) even during deeper stages of anesthesia.5,28 This value of passive PCRIT during anesthesia is similar to the mean value of passive PCRIT values (−4.5 ± 3.0 cm H2O) in normal subjects during natural sleep.6 Therefore, we concluded that tonic neuromuscular activity of upper airway dilator muscles is relatively intact during monitored anesthesia care with midazolam and propofol, and the upper airway mechanical properties are the same as those during sleep. Interestingly, Eikermann et al.49 showed that ketamine is a respiratory stimulant that abolishes the coupling between loss of consciousness and upper airway dilator muscle dysfunction over a wide dose range. They also indicated that ketamine might help stabilize upper airway patency during anesthesia. During sedation or hypnoticinduced sleep, repeated measurements of upper airway collapsibility appear to have less variability, suggesting that arousal and alterations in posture contribute to mechanical alterations in upper airway properties.50Recently, there appears to be a clinical advantage to use dexmedetomidine (DEX) for procedural sedation in pediatric patients and OSA patients.51,52 In contrast to other sedative agents, DEX can provide better sedative properties similar to natural NREM sleep, without major respiratory depression. Therefore, DEX has recently been recommended for sedation during procedural sedation with local anesthesia for children and OSA patients.
Topical anesthesia of the upper airway mucosa, which greatly reduces this reflex response,53,54 causes an increase in upper airway resistance and, thus decreases airflow during sleep. Berry et al. suggested that topical lidocaine applied to the nasal trigeminal area and hypopharynx-laryngeal area markedly induced airflow limitation due to reduction of the amount of phasic activity of the genioglossus electromyogram53 during NREM sleep. A previous study54 also showed that topical anesthesia might increase pharyngeal resistance during stage 1 sleep and at the wake to sleep transitions due to elimination of upper airway mucosal mechanoreceptors. Although the effect of local anesthesia on upper airway collapsibility during anesthesia has not been understood, the influence of local anesthesia in the oro-pharyngeal region may further modify sensitivity to negative airway pressure and chemical reflexes, increasing the patients’ risk during monitored anesthesia care.
HOW CAN UPPER AIRWAY PATENCY BE MAINTAINED? (Figures 5, 6)
It is fundamentally impossible to keep the mouth closed during a procedure in order to maintain upper airway patency by avoiding the effect of mouth opening on the increase in upper airway collapsibility. Therefore, we should establish another mechanical intervention to maintain upper airway patency. How can we minimize the risk of upper airway obstruction during a procedure?Effects of Mandible Advancement on Upper Airway Patency
Previously, we found that mandibular advancement significantly decreased PCRIT to −13.3 ± 3.2 cm H2O (p < 0.05 vs. the centric position), but it did not significantly affect upstream airway resistance (Rua) calculated by equation of VI max = (PN − PCRIT ) / Rua (22.1 ± 6.3 cm H2O/L/s) during midazolam sedation.55 In this study, we evaluated upper airway collapsibility in three different mandibular positions, centric occlusion position, incisors aligned position, and mandible advancement position (75% of the subject’s maximum possible protrusion without any excessive discomfort and pain). Briefly, three different types of rigid-type custom mandible appliance were made during awake condition at different experimental day prior to experimental sedation condition. Three mandibular appliances with centric occlusion position, incisors aligned position, and mandible advancement position (75% of the subject’s maximum possible protrusion) was constructed of clear acrylic resin and 1-mm polyethylene plate (Erkodur; Erkodent Inc.; Pfalzgrafenweiler, Germany) for each subject in reference to previous study by Tsuiki et al.56 This study indicated that mandibular advancement in the incisor-aligned position can decrease both upper airway collapsibility by changes of PCRIT and resistance during midazolam sedation, and that maximal mandible advancement (maximal comfortable protrusion) may not be necessary for the preservation of upper airway patency. We found that mandibular advancement produced isolated decreases in PCRIT, indicating a decrease in collapsibility at the flow-limiting site.28 Moreover, this evidence indicates that mandibular advancement should ameliorate sleep apnea if PCRIT falls by 5 to 10 cm H2O. In patients in whom moderate upper airway obstruction predominates, more modest degrees of mandibular advancement (possibly 25% to 50% of the patient’s maximum possible protrusion) should be clinically effective, since decreases in PCRIT of only 3 to 5 cm H2O are required to relieve airflow obstruction during sleep and sedation. We have also suggested that the degrees of mandibular advancement can be titrated progressively to relieve obstruction in patients with partial or complete upper airway occlusion during sleep,57 possibly due to the allowing muscle fibers adaptation. However, it should be noted that mandible advancement with mouth opening might alter the respiratory phase resetting during swallowing and the timing of swallowing in relation to the respiratory cycle phase. This finding indicates that mandible re-positioning may strongly affect coordination between nasal breathing and nonnutritive swallowing by altering respiratory parameters and by inhibiting movement of the tongue-jaw complex.58
Neck Extension and Chin Lift
Isono et al. reported that neck extension significantly decreased closing pressure of the velopharynx and oropharynx.9 They observed an approximately 3.5-cm H2O reduction in passive PCRIT in the velopharynx and oropharynx and suggested that neck extension significantly decreases compliance of the oropharyngeal airway wall. Previous studies reported that the chin lift caused widening of the entire pharyngeal airway during propofol sedation.59,60 They also suggested that the improvement in airway collapsibility during the chin lift is caused by a combination of increased tension of the pharyngeal muscles and forward movement of the muscles attached to the mandible. A previous study also confirmed that drug-induced sleep endoscopy completed with a simulation bite approach for the prediction of the outcome of treatment of obstructive sleep apnea with mandibular repositioning appliances.61
Sniffing Position (Head Elevation)
Placing the head in the “sniffing position”62 (lower cervical flexion, upper cervical extension with full extension of head on neck) increases longitudinal tension on the upper airway and decreases its collapsibility. Similar to neck extension, the sniffing position increases the distance between the mentum and cervical column, consequently increasing the space enclosed by the maxilla, mandible, and cervical vertebrae. This possibly results in a predictable reduction in passive PCRIT due to improvement of mechanical factors in the sniffing position, although no information is available on changes in soft tissue volume in the pharynx. Recently Kobayashi et al. found a significant reduction in passive PCRIT by a mean value of 4.3 cm H2O in response to 6-cm head elevation during propofol anesthesia with spontaneous breathing.63 They demonstrated that the optimal height of head elevation in normal-weight subjects under propofol anesthesia with spontaneous breathing through the closed mouth was approximately 6.0 cm.
Lateral Position
Boudewyns reported that PCRIT fell from 1.8 cm H2O in the supine position to −1.1 cm H2O (delta 2.9 cm H2O) in the lateral recumbent position.64 Another study found that the upper airway of a spontaneously breathing child who was deeply sedated with propofol widened in the lateral position.65Head Rotation
We previously demonstrated that head rotation decreased upper airway collapsibility in adult subjects during midazolam sedation.66 However, we concluded that the therapeutic effect was insufficient to maintain upper airway patency. A previous study observed that passive PCRIT (−2.8 cm H2O) increased significantly when the head was rotated, compared to PCRIT (−4.4 cm H2O) in the supine condition (delta 1.6 cm H2O) in pediatric patients, indicating a significant increase in pharyngeal airway collapsibility in the head rotated position.67Upper Body Elevation (Sitting Position)
A previous study68 found that a 30-degree elevation of the upper body resulted in an improvement of upper airway collapsibility compared with both the supine and lateral positions, as seen by measuring upper airway closing pressures in patients with obstructive sleep apnea. They reported that a 30-degree elevation caused a 4.3-cm H2O decrease in PCRIT, while adopting the lateral position caused a 1.4-cm H2O decrease in PCRIT relative to the supine position. In our previous study,66 we found a 5.4-cm H2O decrease in closing pressure after 30-degree upper body elevation compared to the supine position. A previous study indicated that postural change from supine to sitting positions enlarged both retropalatal and retroglossal airways and decreased PCRIT in both pharyngeal segments by approximately 6 cm H2O in completely paralyzed and anesthetized patients with OSA.69 They postulated that this improvement can be due to mechanical interaction between the thorax and upper airway, such that caudal movement of the larynx with increasing lung volume results in secondary stiffening and dilatation of the pharynx.69
Figure 5Effects of head and upper body position on upper airway collapsibility during sedation. |
Figure 6Predicted effects of mechanical intervention on changes in critical closing pressure (PCRIT) in obese patients during sedation. |
CONCLUSION
Mechanical upper airway anatomy may become the dominant factor governing upper airway collapsibility during sleep and sedation due to the significant impairment of neural mechanisms controlling compensatory neuromuscular responses. It is, therefore, important to understand the effectiveness of mechanical interventions and develop a systematic approach to evaluating the factors that contribute to maintenance of upper airway patency during sleep and sedation.REFERENCES
2. Hillman D, Singh B, McArdle N, Eastwood P. Relationships between ventilatory impairment, sleep hypoventilation and type 2 respiratory failure. Respirology 2014;19:1106–16.
3. Horner RL, Hughes SW, Malhotra A. State-dependent and reflex drives to the upper airway: basic physiology with clinical implications. J Appl Physiol 2014;116:325–36.
4. Haeseler G, Stormer M, Bufler J, et al. Propofol blocks human skeletal muscle sodium channels in a voltage-dependent manner. Anesth Analg 2001;92:1192–8.
5. Hoshino Y, Ayuse T, Kurata S, et al. The compensatory responses to upper airway obstruction in normal subjects under propofol anesthesia. Respir Physiol Neurobiol 2009;166:24–31.
6. Patil SP, Schneider H, Marx JJ, Gladmon E, Schwartz AR, Smith PL. Neuromechanical control of upper airway patency during sleep. J Appl Physiol 2007;102:547–56.
7. Isono S, Remmers JE, Tanaka A, Sho Y, Sato J, Nishino T. Anatomy of pharynx in patients with obstructive sleep apnea and in normal subjects. J Appl Physiol 1997;82:1319–26.
8. Remmers JE, deGroot WJ, Sauerland EK, Anch AM. Pathogenesis of upper airway occlusion during sleep. J Appl Physiol 1978;44:931–8.
9. Isono S, Tanaka A, Tagaito Y, Ishikawa T, Nishino T Influences of head positions and bite opening on collapsibility of the passive pharynx. J Appl Physiol 2004;97:339–46.
10. King ED, O’Donnell CP, Smith PL, Schwartz AR A model of obstructive sleep apnea in normal humans. Role of the upper airway. Am J Respir Crit Care Med 2000;161:1979–84.
11. Schneider H, Patil SP, Canisius S, et al. Hypercapnic duty cycle is an intermediate physiological phenotype linked to mouse chromosome 5. J Appl Physiol 2003;95:11–9.
12. Tagaito Y, Schneider H, O’Donnell CP, Smith PL, Schwartz AR. Ventilating with tracheal gas insufflation and periodic tracheal occlusion during sleep and wakefulness. Chest 2002;122:1742–50.
13. Schneider H, Schwartz AR, Smith PL, Patil SP, Krishnan V, Pichard L. Duty cycle responses to flow limitation predict nocturnal hypoventilation. Eur Respir J 2008;32:255–8.
14. Ayuse T, Hoshino Y, Kurata S, et al. The effect of gender on compensatory neuromuscular response to upper airway obstruction in normal subjects under midazolam general anesthesia. Anesth Analg 2009;109:1209–18.
15. Eckert DJ, Catcheside PG, McDonald R, et al. Sustained hypoxia depresses sensory processing of respiratory resistive loads. Am J Respir Crit Care Med 2005;172:1047–54.
16. Cortelli P, Lombardi C, Montagna P, Parati G. Baroreflex modulation during sleep and in obstructive sleep apnea syndrome. Auton Neurosci 2012;169:7–11.
17. Gold AR, Schwartz AR. The pharyngeal critical pressure. The whys and hows of using nasal continuous positive airway pressure diagnostically. Chest 1996;110:1077–88.
18. Schwartz AR, Smith PL, Wise RA, Bankman I, Permutt S. Effect of positive nasal pressure on upper airway pressure-flow relationships. J Appl Physiol 1989;66:1626–34.
19. Wei T, Erlacher MA, Grossman P, et al. Approach for streamlining measurement of complex physiological phenotypes of upper airway collapsibility. Comput Biol Med 2013;43:600–6.
20. Joosten SA, O’Driscoll DM, Berger PJ, Hamilton GS. Supine position related obstructive sleep apnea in adults: pathogenesis and treatment. Sleep Med Rev 2014;18:7–17.
21. Shepard JW, Jr., Pevernagie DA, Stanson AW, Daniels BK, Sheedy PF. Effects of changes in central venous pressure on upper airway size in patients with obstructive sleep apnea. Am J Respir Crit Care Med 1996;153:250–4.
22. Su MC, Chiu KL, Ruttanaumpawan P et al. Lower body positive pressure increases upper airway collapsibility in healthy subjects. Respir Physiol Neurobiol 2008;161:306–12.
23. Kasai T, Motwani SS, Elias RM, et al. Influence of rostral fluid shift on upper airway size and mucosal water content. J Clin Sleep Med 2014;10:1069–74.
24. White LH, Lyons OD, Yadollahi A, Ryan CM, Bradley TD. Night-tonight variability in obstructive sleep apnea severity: relationship to overnight rostral fluid shift. J Clin Sleep Med 2015;11:149–56.
25. Rosenfeld R, Livne D, Nevo O, et al. Hormonal and volume dysregulation in women with premenstrual syndrome. Hypertension 2008;51:1225–30.
26. Walsh JH, Maddison KJ, Platt PR, Hillman DR, Eastwood PR. Influence of head extension, flexion, and rotation on collapsibility of the passive upper airway. Sleep 2008;31:1440–7.
27. Boidin MP. Airway patency in the unconscious patient. Br J Anaesth 1985;57:306–10.
28. Ayuse T, Inazawa T, Kurata S, et al. Mouth-opening increases upperairway collapsibility without changing resistance during midazolam sedation. J Dent Res 2004;83:718–22.
29. Meurice JC, Marc I, Carrier G, Series F. Effects of mouth opening on upper airway collapsibility in normal sleeping subjects. Am J Respir Crit Care Med 1996;153:255–9.
30. Bonuck K, Parikh S, Bassila M. Growth failure and sleep disordered breathing: a review of the literature. Int J Pediatr Otorhinolaryngol 2006;70:769–78.
31. Soultan Z, Wadowski S, Rao M, Kravath RE. Effect of treating obstructive sleep apnea by tonsillectomy and/or adenoidectomy on obesity in children. Arch Pediatr Adolesc Med 1999;153:33–7.
32. Mallory GB Jr., Fiser DH, Jackson R. Sleep-associated breathing disorders in morbidly obese children and adolescents. J Pediatr 1989;115:892–7.
33. Singhal P, Gupta R, Sharma R, Mishra P. Association of naso-oropharyngeal structures with the sleep architecture in suspected obstructive sleep apnea. Indian J Otolaryngol Head Neck Surg 2014;66:81–7.
34. Chin CJ, Khami MM, Husein M. A general review of the otolaryngologic manifestations of Down Syndrome. Int J Pediatr Otorhinolaryngol 2014;78:899–904.
35. Ferguson KA, Love LL, Ryan CF. Effect of mandibular and tongue protrusion on upper airway size during wakefulness. Am J Respir Crit Care Med 1997;155:1748–54.
36. Pae EK, Lowe AA, Sasaki K, Price C, Tsuchiya M, Fleetham JA. A cephalometric and electromyographic study of upper airway structures in the upright and supine positions. Am J Orthod Dentofacial Orthop 1994;106:52–9.
37. Lowe AA. The tongue and airway. Otolaryngol Clin North Am 1990;23:677–98.
38. Hoshino Y, Ayuse T, Kobayashi M, et al. The effects of hormonal status on upper airway patency in normal female subjects during propofol anesthesia. J Clin Anesth 2011;23:527–33.
39. Chang CT, Sun CY, Pong CY, et al. Interaction of estrogen and progesterone in the regulation of sodium channels in collecting tubular cells. Chang Gung Med J 2007;30:305–12.
40. Su MC, Chiu KL, Ruttanaumpawan P, et al. Difference in upper airway collapsibility during wakefulness between men and women in response to lower-body positive pressure. Clin Sci (Lond) 2009;116:713–20.
41. Lam JC, Kairaitis K, Verma M, Wheatley JR, Amis TC. Saliva production and surface tension: influences on patency of the passive upper airway. J Physiol 2008;586:5537–47.
42. Kirkness JP, Eastwood PR, Szollosi I, et al. Effect of surface tension of mucosal lining liquid on upper airway mechanics in anesthetized humans. J Appl Physiol 2003;95:357–63.
43. Kawai M, Kirkness JP, Yamamura S, et al. Increased phosphatidylcholine concentration in saliva reduces surface tension and improves airway patency in obstructive sleep apnoea. J Oral Rehabil 2013;40:758–66.
44. Usmani ZA, Hlavac M, Rischmueller M, et al. Sleep disordered breathing in patients with primary Sjogren’s syndrome: a group controlled study. Sleep Med 2012;13:1066–70.
45. Hilditch CJ, McEvoy RD, George KE, et al. Upper airway surface tension but not upper airway collapsibility is elevated in primary Sjogren’s syndrome. Sleep 2008;31:367–74.
46. Eastwood PR, Platt PR, Shepherd K, Maddison K, Hillman DR. Collapsibility of the upper airway at different concentrations of propofol anesthesia. Anesthesiology 2005;103:470–7.
47. Norton JR, Ward DS, Karan S, et al. Differences between midazolam and propofol sedation on upper airway collapsibility using dynamic negative airway pressure. Anesthesiology 2006;104:1155–64.
48. Hillman DR, Walsh JH, Maddison KJ, et al. Evolution of changes in upper airway collapsibility during slow induction of anesthesia with propofol. Anesthesiology 2009;111:63–71.
49. Eikermann M, Grosse-Sundrup M, Zaremba S, et al. Ketamine activates breathing and abolishes the coupling between loss of consciousness and upper airway dilator muscle dysfunction. Anesthesiology 2012;116:35–46.
50. Kirkness JP, Peterson LA, Squier SB, et al. Performance characteristics of upper airway critical collapsing pressure measurements during sleep. Sleep 2011;34:459–67.
51. Nelson LE, Lu J, Guo T, Saper CB, Franks NP, Maze M. The alpha2- adrenoceptor agonist dexmedetomidine converges on an endogenous sleep-promoting pathway to exert its sedative effects. Anesthesiology 2003;98:428–36.
52. Mahmoud M, Jung D, Salisbury S, et al. Effect of increasing depth of dexmedetomidine and propofol anesthesia on upper airway morphology in children and adolescents with obstructive sleep apnea. J Clin Anesth 2013;25:529–41.
53. Berry RB, McNellis MI, Kouchi K, Light RW. Upper airway anesthesia reduces phasic genioglossus activity during sleep apnea. Am J Respir Crit Care Med 1997;156:127–32.
54. Doherty LS, Nolan P, McNicholas WT. Effects of topical anesthesia on upper airway resistance during wake-sleep transitions. J Appl Physiol 2005;99:549–55.
55. Inazawa T, Ayuse T, Kurata S, et al. Effect of mandibular position on upper airway collapsibility and resistance. J Dent Res 2005;84:554–8.
56. Tsuiki S, Ono T, Kuroda T. Mandibular advancement modulates respiratory-related genioglossus electromyographic activity. Sleep Breath 2000;4:53–58.
57. Ayuse T, Hoshino Y, Inazawa T, Oi K, Schneider H, Schwartz AR. A pilot study of quantitative assessment of mandible advancement using pressure-flow relationship during midazolam sedation. J Oral Rehabil 2006;33:813–9.
58. Ayuse T, Ishitobi S, Yoshida H, et al. The mandible advancement may alter the coordination between breathing and the non-nutritive swallowing reflex. J Oral Rehab 2010;37:336–45.
59. Shorten GD, Armstrong DC, Roy WI, Brown L. Assessment of the effect of head and neck position on upper airway anatomy in sedated paediatric patients using magnetic resonance imaging. Paediatr Anaesth 1995;5:243–8.
60. Reber A, Wetzel SG, Schnabel K, Bongartz G, Frei FJ. Effect of combined mouth closure and chin lift on upper airway dimensions during routine magnetic resonance imaging in pediatric patients sedated with propofol. Anesthesiology 1999;90:1617–23.
61. Vanderveken OM, Vroegop AM, Van de Heining PH, Braem MJ. Druginduced sleep endoscopy completed with a simulation bite approach for the prediction of the outcome of treatment of obstructive sleep apnea with mandibular repositioning appliances. Open Tech Otolaryngol 2011;22:175–82.
62. Isono S, Tanaka A, Ishikawa T, Tagaito Y, Nishino T. Sniffing position improves pharyngeal airway patency in anesthetized patients with obstructive sleep apnea. Anesthesiology 2005;103:489–94.
63. Kobayashi M, Ayuse T, Hoshino Y, et al. Effect of head elevation on passive upper airway collapsibility in normal subjects during propofol anesthesia. Anesthesiology 2011;115:273–81
64. Boudewyns A, Punjabi N, Van de Heyning PH, et al. Abbreviated method for assessing upper airway function in obstructive sleep apnea. Chest 2000;118:1031–41.
65. Litman RS, Wake N, Chan LM, et al. Effect of lateral positioning on upper airway size and morphology in sedated children. Anesthesiology 2005;103:484–8.
66. Ikeda H, Ayuse T, Oi K. The effects of head and body positioning on upper airway collapsibility in normal subjects who received midazolam sedation. J Clin Anesth 2006;18:185–93.
67. Ishikawa T, Isono S, Aiba J, Tanaka A, Nishino T. Prone position increases collapsibility of the passive pharynx in infants and small children. Am J Respir Crit Care Med 2002;166:760–4.
68. Neill AM, Angus SM, Sajkov D, McEvoy RD. Effects of sleep posture on upper airway stability in patients with obstructive sleep apnea. Am J Respir Crit Care Med 1997;155:199–204.
69. Tagaito Y, Isono S, Tanaka A, Ishikawa T, Nishino T. Sitting posture decreases collapsibility of the passive pharynx in anesthetized paralyzed patients with obstructive sleep apnea. Anesthesiology 2010;113:812–8.
SUBMISSION & CORRESPONDENCE INFORMATION
Submitted for publication April, 2015
Submitted in final revised form September, 2015
Accepted for publication September, 2015
Address correspondence to: Takao Ayuse, Division of Clinical Physiology, Course of Medical and Dental Sciences, Department of Translational Medical Sciences, Nagasaki University Graduate School of Biomedical Sciences, 1-7-1 Sakamoto, Nagasaki 852-8588, Japan; Tel: +81-95-819-7714; Fax: +81-95-819-7715; Email: ayuse@nagasaki-u.ac.jp
DISCLOSURE STATEMENT
This was not an industry supported study. The study was funded in part by the funds from a contributors’ institution and Grants-in-Aid for Scientific Research from Japan Society for the Promotion Science (JSPS). (5-3-1 Kojimachi, Chiyoda-ku, Tokyo Japan). The authors have indicated no financial conflicts of interest.
PDF