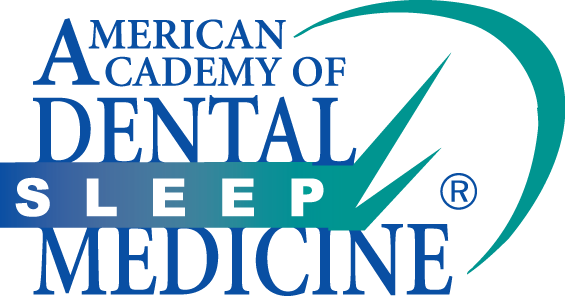
Original Article 2, Issue 11.3
The Effect of Mandibular Advancement Devices on Sympathetic Nerve Activity and Markers of Cardiovascular Health in Obstructive Sleep Apnea Patients: A Prospective Case Series Protocol
http://dx.doi.org/10.15331/jdsm.7344Emily King, MSc1, Sana Ayesha, MBBS2, Craig Steinback, PhD3, Carlos Flores-Mir, DSc4*
1 Private Practice in Medicine Hat, AB, Canada, 2 MSc student, Faculty of Kinesiology, Sport, and Recreation, University of Alberta, Edmonton, AB, Canada, 3 Professor, Faculty of Kinesiology, Sport, and Recreation, University of Alberta, Edmonton, AB, Canada, 4 Professor, Faculty of Medicine and Dentistry, University of Alberta, Edmonton, AB, Canada
ABSTRACT
Study Objectives:
The effect of mandibular advancement devices (MADs) on sympathetic nerve activity (SNA) has not been measured directly through microneurography. Therefore, this protocol aims to determine whether MADs improve SNA, vascular health, blood pressure, and indirect markers of SNA (heart rate variability and concentrations of neurotransmitters in the blood) in adult study participants with OSA.
Methods:
Patients with a diagnosis of OSA will be referred by multiple dental practitioners in Edmonton, Alberta who are certified to provide MAD therapy. A sample size of 50 participants is planned, considering a 20% dropout rate. Participants will be examined at baseline and again after 3 and 6 months of efficacious MAD therapy. The following outcomes will be recorded at each time point: direct SNA via microneurography, heart rate, continuous blood pressure, flow-mediated dilation (a marker of vascular health), blood concentration of noradrenaline, apnea-hypopnea index (AHI) via a level 3 take-home sleep study, and MAD compliance via a scorecard for self-reported nighttime wear.
Clinical Implications:
Understanding how direct and indirect measures of SNA relate to OSA therapy may be valuable, as some participants and researchers consider microneurography an invasive and complex technique. Improved SNA and vascular health would further support MADs as an important alternative to no treatment. Finally, research examining the effect of various OSA therapies on the sympathetic influence of blood pressure and the heart is critical to understanding how changes in cardiac autonomic modulation may influence cardiovascular risk.
Keywords:
Cardiovascular health, endothelial dysfunction, mandibular advancement device, obstructive sleep apnea, sympathetic nervous activity.
Citation:
King E, Ayesha S, Steinback C, et al. The Effect of Mandibular Advancement Devices on Sympathetic Nerve Activity and Markers of Cardiovascular Health in Obstructive Sleep Apnea Patients: A Prospective Case Series Protocol. J Dent Sleep Med. 2024;11(3)
INTRODUCTION
Obstructive sleep apnea (OSA) is a type of disordered breathing defined by repetitive airflow obstruction during sleep due to upper airway collapse. The obstruction can be either partial (hypopnea) or complete (apnea), and each obstructive event contributes to decreased blood oxygen (ie, hypoxia). The severity of OSA has been mainly determined by the apnea-hypopnea index (AHI), which is the average number of airway obstructions experienced per hour of sleep. In middle-aged populations, it is estimated that 17% of women and 34% of men meet the diagnostic criteria for OSA.1 OSA has been associated with various cardiovascular diseases, including hypertension, stroke, heart failure, and coronary artery disease.2 A factor in this association may be the decrease in blood vessel health and the marked overactivation of the sympathetic nervous system (SNS) observed in OSA due to intermittent hypoxia.3-6
The SNS and the parasympathetic nervous system are the two branches of the autonomic nervous system responsible for maintaining cardiovascular balance. The SNS innervates the heart and peripheral vasculature and responds to changes in blood pressure via baroreceptors and alterations in blood gases such as oxygen and carbon dioxide via chemoreceptors.7 Autonomic responses to intermittent airway collapse in OSA are multifactorial, involving the effects of hypoxia, hypercapnia, inspiration against an obstructed airway, and arousal from sleep. These mechanisms each act uniquely to elevate sympathetic nerve activity (SNA) during apneic episodes.8 Autonomic abnormalities are speculated to link OSA with hypertension and cardiovascular morbidity.9 Research has found daytime SNA is elevated even in patients with mild, untreated OSA.10 In addition, AHI has been positively correlated with SNA, suggesting a relationship between autonomic abnormalities and OSA severity.11
Continuous positive airway pressure (CPAP) is an effective treatment for OSA and has been shown to improve patients' blood pressure, AHI, and SNA.12-14 However, the benefits are dose-dependent and rely on patient compliance. Unfortunately, 30% to 40% of patients are nonadherent to CPAP therapy over the long term.15 Mandibular advancement devices (MAD) are an accepted alternative treatment for OSA. Despite their decreased efficacy in lowering AHI, they show similar reductions in blood pressure when compared with CPAP. They are suggested to improve disease progression through increased compliance compared with CPAP.16,17 A 2013 cohort study of 570 patients found that in comparison with patients with severe OSA who underwent CPAP or MAD treatment, untreated patients had a six times higher risk of dying from a cardiovascular event (2 per every 100 patients). Additionally, there was no difference in cardiovascular death rate between MAD and CPAP-treated participants.18 Therefore, understanding the effect of alternative therapies to CPAP on the progression of OSA via changes in AHI and cardiovascular markers is paramount. However, AHI has been criticized for being a poor proxy of nocturnal oxygen desaturation because it fails to quantify the depth and duration of each hypoxic event.19 This is an essential consideration, because sleep-disordered breathing and low nocturnal oxygen desaturation have been identified as independent risk factors for sudden cardiac death.20-22 In contrast, SNA is known to respond acutely and chronically to hypoxia.23
Studies investigating changes in SNA during MAD therapy have been attempted using only indirect measures, such as heart rate variability (HRV). HRV is calculated from an electrocardiogram (ECG), with the time variation between each heartbeat reflecting the balance between the SNS and parasympathetic nervous system, or cardiac autonomic modulation.24 Another promising indirect measurement is the use of skin sympathetic activity (SKNA) in patients with OSA.25,26
A 2018 systematic review and meta-analysis by de Vries et al16 investigated the effects of oral appliance therapy on a wide range of cardiovascular outcomes. It was reported that only four studies, two of them randomized controlled trials, have researched the effect of MAD therapy on HRV. The study authors concluded that 3 months of MAD therapy appears to have some small favorable effect on HRV. However, sample sizes are small, and results are somewhat heterogeneous because varying HRV analyses and parameters have been used to support this conclusion. Furthermore, conclusions could not extend the understanding of the SNS, because the current consensus is that the HRV relates most clearly to parasympathetic processes, but not necessarily to sympathetic processes.23, 24, 27 A meta-analysis of HRV was not possible because of differences in units used for HRV parameters.16 Regarding SKNA in patients with OSA, studies have shown that average SKNA is reduced, SKNA frequency shifts, and not all patients with OSA have increased sympathetic tone. 25,26 Therefore, the SNS response to MAD therapy currently needs to be better defined and understood.
Difficulties in accurately assessing the human SNS have been noted in the literature. Although no gold standard technique exists, the preferred assessment method is microneurography along with noradrenaline spillover analysis.27 Microneurography is the only method that directly records efferent postganglionic muscle sympathetic nerve activity in real time as vasoconstricting impulses are sent to the periphery to regulate blood pressure. This is accomplished by inserting a tungsten microelectrode into a nerve fascicle.28 Substantial and consistent evidence for altered SNA in OSA has come from microneurography, which has been established as an effective tool for measuring acute and chronic changes in SNA.10-12, 27 Yet, most studies use HRV as a primary indicator for SNA because of its relative simplicity.29 No studies are known to directly measure SNA in a MAD intervention with microneurography. Understanding how direct and indirect measures of SNA relate to OSA therapy may be valuable as microneurography is considered an invasive and complex technique to perform by some participants and researchers.30,31 Improved SNA and vascular health would further support MADs as an important alternative to no treatment. Finally, research examining the effect of various OSA therapies on the sympathetic influence of blood pressure and the heart is critical to understanding how changes in cardiac autonomic modulation may influence cardiovascular risk.
METHODS AND ANALYSIS
This study was approved by the Health Research Ethics Board-Health Panel, University of Alberta, Edmonton, Canada (Pro00108618).
Study Design
A prospective self-controlled case series study is planned to evaluate the effect of MAD therapy in participants with OSA diagnosed using direct and indirect SNA measures, vascular health, and other cardiovascular outcomes, including blood pressure and heart rate. A repeated-measures design was chosen because of the large number of factors that can cause individual variability in SNA and vascular health. Additionally, using microneurography to obtain SNA outcomes is a highly technical procedure requiring training and resources. Therefore, a self-controlled repeated- measures study design allows fewer recruitment requirements, making the study more efficient.Sample Definition and Eligibility Criteria
Participants who fit the following inclusion criteria will be permitted to participate: (1) diagnosis of OSA by a physician; (2) prescription of a titratable MAD from a certified dentist who has followed the standard of care guidelines for appropriate patient selection and multidisciplinary patient management; (3) age 18 years or older; (4) AHI greater than 5 events per hour; (5) body mass index less than 40 kg/m2.
Participants will be excluded from participation for the following reasons: (1) an inability to breathe comfortably through the nose; (2) poor pulmonary function; (3) failure to obtain an OSA diagnosis from a physician; (4) diagnosis of central sleep apnea or mixed apnea; (5) anticipated medical changes that could alter the severity of OSA; (6) expected change in body weight by >5% during the study; (7) preexisting symptomatic nonrespiratory sleep disorder (eg, restless leg syndrome, chronic insomnia); (8) smoking habit; or (9) prescription of a nontitrable appliance.
Recruitment and Sample Size Rationale
Dental practitioners who adhere to the current standard of care guidelines outlined by the College of Dental Surgeons of Alberta for providing MADs will be approached to refer potential participants to this study. This referral process will rely on the clinical judgment of the referring dental professional to select patients for whom the MADs will be effective. To help ensure MAD efficacy, only patients who are prescribed adjustable MADs and agree to adhere to the titration protocol outlined by their dentist will be approached to participate in the study. Participants deemed unsuccessful in MAD therapy by their dental practitioner will not be retested after baseline.
A 2022 systematic review and meta-analysis revealed inconclusive data regarding the optimal length of intervention for producing a significant change in SNA. Changes in SNA were detected by microneurography after 1 month of CPAP use, with similar changes detected after 12 months of CPAP use.11 Several prospective studies measuring changes in SNA indirectly through HRV found small positive changes in cardiac autonomic modulation after 3 months of MAD intervention in patients with mild to severe OSA.32-35 Therefore, in the current study, participants with successful MAD titration as per their dental practitioner will be tested after 3 and 6 months of MAD use after reaching device efficacy. Analysis of the difference between SNA at T0, T1, and T2 considering alpha = 0.05, statistical power = 0.9, and medium-large effect size (0.7) will require an estimated minimum sample size of 44. A sample size of 50 patients will be appropriate, considering a 20% dropout rate. An interim analysis is proposed, after 10 participants have completed the protocol to calculate the final sample size more accurately.
Primary Outcome
The primary outcome of this study is the effect of 3 and 6 months of efficacious MAD therapy on SNA in patients with mild to severe OSA as measured directly through muscle sympathetic nerve activity (MSNA) microneurography.
Secondary Outcome
The secondary outcome of this study is the effect of 3 and 6 months of efficacious MAD therapy on vascular health as assessed through flow-mediated dilation (FMD).
Other Outcomes
The results of two indirect SNA measurements, HRV and blood noradrenaline concentration analysis, will be explored compared with direct measurement of SNA through microneurography, which is considered a more accurate technique for assessing the SNS.27 Self-reported MAD wear (in hours per night) and posttreatment AHI will also be recorded to quantify therapy response for analysis and potential subgroup analysis of responders versus nonresponders. Responders will be defined as those who achieve a reduction in AHI by more than 50% and a final AHI of less than 10 events per hour compared with baseline.36 Participant age, body mass index, pretreatment AHI, neck circumference, and millimeters of mandibular advancement will also be recorded.
LABORATORY PROTOCOL
After the initial screening and confirmation of written informed consent by a trained researcher, participants arrive at the laboratory for baseline testing before beginning MAD therapy. They will come in the morning after a light breakfast (eg, toast and water) and abstain from caffeine, alcohol, and strenuous exercise for 12 hours. Female participants with a regular menstrual cycle will be tested during the early follicular phase of the menstruation cycle. Female participants taking oral contraceptives or using intrauterine hormonal devices will be tested at their convenience. Subsequent testing sessions at 3 and 6 months of efficacious MAD wear will be completed simultaneously and under the same conditions as outlined previously.
In the laboratory, participants will be seated comfortably in a dental chair reclined 45°, and a blood sample will be obtained to measure the concentration of the neurotransmitter noradrenaline in the blood. Next, a standard lead II ECG (ML 132, ADInstruments, Colorado Springs, CO, USA) will be used to record participants’ heart rate, and a blood pressure cuff and finger cuff will be used to record beat-by-beat by finger pulse photoplethysmography (Finometer Pro, Finapres Medical Systems, the Netherlands). All cardiovascular parameters will be acquired using an analog-to-digital converter (Powerlab/16SP ML 880; ADInstruments, Colorado Springs, CO, USA) interfaced with a personal computer. Commercially available software will analyze cardiovascular variables (LabChart V7.1, ADinstruments, Colorado Springs, CO, USA).
Next, a trained technician will record the multiunit postganglionic MSNA via microneurography using a sterile tungsten recording microelectrode (35 mm long, 200 μm in diameter, tapered to a 1- to 5-μm uninsulated tip), which will be inserted into a muscle-nerve fascicle of a sympathetic nerve bundle of the common peroneal nerve (Figure 1). A reference electrode will also be inserted subcutaneously 1 to 3 cm from the recording electrode. MSNA will be obtained by manually manipulating the microelectrode until the following criteria are confirmed: (1) pulse synchronous activity; (2) sympathoexcitatory response to end-expiratory apnea; and (3) no response to skin stroking or startle stimuli (eg, sudden shout). Raw nerve signals are amplified (1000× preamplifier and 100× variable gain isolated amplifier), band-pass filtered (700-2,000Hz), rectified, and integrated (constant decay 0.1s) to obtain an integrated voltage neurogram (model 662C-3; Iowa University Bioengineering, Iowa City, IA, USA). MSNA data will be sampled at 10,000 Hz and stored for offline analysis in LabChart (Powerlab Software, ADInstruments, Chart Pro v8.3.1, Colorado Springs, CO).
Following instrumentation, participants will rest quietly for 5 minutes to relax. Next, MSNA, continuous blood pressure, and heart rate data will be recorded for 10 minutes. After 10 minutes, a blood vessel health test (FMD) will be completed. This test represents the production and the bioavailability of a potent blood vessel-derived relaxing factor, nitric oxide, that stimulates vasodilation and provides an excellent indicator of vascular health. This will be assessed in the brachial artery using a sphygmomanometer cuff and a linear array probe attached to a Duplex ultrasound machine (12MHz linear array probe, GE Vivid 7; DV12USB, Epiphan Systems, Mississauga, Ontario, Canada) at an insonation angle of 60°. The sphygmomanometer cuff will be placed on the forearm, and then baseline brachial artery blood flow velocity and diameter will be recorded by an experienced sonographer for 1 minute. Next, the cuff will be inflated to a supra-systolic pressure (250 mmHg) to occlude forearm blood flow for 5 min. Then, the cuff will be rapidly deflated (approximately 1 s) while the sonographer continues to record changes in brachial blood velocity and diameter (Figure 2). Participant preparation will be completed per the suggested guidelines by Thijssen et al.37
Once testing is completed, the participant will be sent home with a level 3 sleep study to use overnight (ApneaLink Air, ResMed, Sydney, Australia). This will allow the categorization of participants by AHI response for later analysis and discussion. The participant will also be given a scorecard to track the approximate number of hours the MAD is worn to screen for non-compliance. Compliance will be defined as wearing the MAD for a minimum of 80% per night, 5 or more nights per week.38
Microneurography
Microneurography is a direct method of assessing the sympathetic outflow to the vasculature of the skin and muscle. The study protocol focuses on MSNA, which consists of efferent vasoconstrictor impulses to the large peripheral vascular bed in the body’s skeletal muscle. Through constricting skeletal muscle, MSNA critically influences blood flow and resistance in the vasculature and plays a critical role in blood pressure regulation and hemodynamic stability.3 MSNA demonstrates a high intraindividual reproducibility even over years, which allows for long-term monitoring of disease processes and therapeutic interventions.37 However, MSNA has high interindividual variability and is additionally influenced by short-term factors such as eating a large meal, maintaining a full bladder, mental stress, and changes in posture or breathing cadence. Long-term modifiers of MSNA include aging, sex and female hormonal cycles, genetics, and obesity. Therefore, the careful study design is critical in keeping short-term factors consistent during repeated measures and considering long-term factors in recruitment and data analysis.3
Flow-mediated Dilation
Endothelial dysfunction can predict the development of atherosclerosis and vascular disease and is a marker of cardiovascular risk. FMD is the most used test to measure an artery's capacity to dilate by releasing nitric oxide in response to stimulating endothelial cells by reactive hyperemia.40 FMD is a highly reproducible measure, even among testing centers, if standardized criteria for participant preparation are followed.39 However, patients of older age and those with hypertension or dyslipidemia have more significant variability in FMD repeated measures.37
Blood Concentrations of Noradrenaline
Measuring noradrenaline spillover from sympathetic nerve impulses into the circulation indirectly assesses overall SNS activity. A significant positive correlation has been established between noradrenaline plasma concentration and microneurography measures of SNA in humans at rest.41 However, interpretation is sometimes complicated because plasma noradrenaline is the net result of many factors, including noradrenaline clearance, metabolism, excretion, and total plasma volume. Therefore, plasma noradrenaline concentrations may not accurately reflect SNA in every situation.42
Heart rate variability
HRV indirectly measures cardiac autonomic balance via power spectral analysis of an ECG. This technique produces several mathematical parameters describing time and frequency component variations within each heartbeat cycle. The amount of variation detected reflects the ability of the autonomic nervous system to maintain cardiovascular balance. HRV best describes parasympathetic nerve activity, and its ability to assess SNA accurately is heavily debated in the literature.23 Oversimplification during interpretation can lead to unfounded conclusions if standardized protocols are not followed.24 However, AHI has been negatively correlated with HRV, suggesting a relationship with OSA severity.6
Figure 1A microneurography recording from a tungsten microelectrode. The right of the image depicts the resultant filtered and integrated neurograms. |
Figure 2Ultrasound images showing post-occlusion changes in brachial arterial dimension and blood flow after forearm cuff release during flow-mediated dilation test. |
DATA ANALYSIS PLAN
Direct and indirect SNA and FMD values collected at 3 and 6 months will be compared with baseline values for self-controlled comparisons. All LabChart and FMD video files will be assigned a random identifier before analysis to blind the analyzing researcher from participant identification and time point. A repeated-measures multivariate analysis of covariance will be used to assess statistical differences between the mean of the dependent variables AHI, oxygen desaturation index, cardiovascular variables, and mean percent change in MSNA variables (burst frequency and burst incidence) and FMD (normalized percent change) after 3 months of treatment and 6 months of treatment versus baseline. Body mass index, neck circumference, sex, and age will be covariates. Within-subject factors will include time (three levels) to address primary question and SNA measurement method (three levels: direct, microneurography, and indirect; HRV and blood noradrenaline) to address tertiary question of whether indirect methods produce comparable conclusions to direct methods.
Analysis 1
The effect of MAD therapy on MSNA will be determined by identifying and quantifying bursts of sympathetic activity from the integrated neurogram obtained during data collection. Bursts will be initially identified using a semiautomated peak detection algorithm and confirmed by visual inspection by a trained observer using established criteria by Meah et al,31 including peak morphology, relation to diastolic blood pressure, and 3:1 signal-to-noise ratio. Sympathetic activity will then be quantified as burst frequency (bursts/min), burst incidence (bursts/100 heartbeats), burst amplitude (normalized to largest resting amplitude), and total activity (burst frequency multiplied by mean normalized burst amplitude). A decrease in these quantifications will indicate a positive change in MSNA. If accompanied by a reduction in AHI, sufficient self-reported MAD compliance, and a nonsignificant change in short-term influencing factors, an association between successful MAD therapy and decreased MSNA may be detected.
Analysis 2
The effect of MAD therapy on vascular health will be determined through FMD analysis. Baseline arterial diameter (DA – arterial diameter) will be compared with the peak postocclusion DA expressed as the change in arterial diameter (FMD) as well as percent change (FMD%). FMD values will be normalized to vascular shear stress stimulus, calculated as the product of shear rate and blood viscosity leading to peak arterial diameter. Blood viscosity will be measured at a shear rate of 225s-1 using a cone-plate rheometer (DVNext Rheometer, Brookfield Ametek, Middleboro, MA, USA) at 36°C for 1 minute. The shear rate will be calculated as 8A/A; where A is arterial blood velocity.37 Brachial artery diameter and blood velocity measurements will be obtained from offline ultrasound analysis (Brachial Analyzer, Medical Imaging Applications; qDAT, Coralville, IA). Forearm vascular conductance will be calculated as blood flow velocity divided by mean arterial blood pressure. If a decrease in AHI accompanies an increase in normalized FMD%, an association between successful MAD therapy and improved vascular health may be detected.
Analysis 3
HRV analysis will be conducted on 5 minutes of artifact-free ECG data to obtain the time-domain parameters RRI (ms), SDNN (ms), RMSSD (ms) and the frequency-domain parameters total power (TP; ms2), very low frequency (VLF; ms2: approximately <0.04 Hz), low frequency (LF; ms2; range 0.01-0.15 Hz), high frequency (HF; ms2; range 0.15-0.4 Hz), low frequency normalized units (LFnu; LF/(TP-VLF) x 100), and high frequency normalized units (HFnu; HF/(TP-VLF) x 100). Calculations and interpretations will follow the recommendations outlined in the European Society of Cardiology and the North American Society of Pacing and Electrophysiology 1996 Task Force paper on HRV best practices and standards.44 Doing so will allow comparison of these findings with other works following the same guidelines, as HRV following dissimilar analyses is otherwise incomparable.
Blood samples will be analyzed for noradrenaline concentrations in nanomoles per liter (nmol/L) as an additional indirect measure of SNA. Concentrations of blood noradrenaline and HRV parameters at 3 and 6 months will be compared against baseline values. Correlations between direct and indirect measures will be explored. Potential differences in significance among direct and indirect findings will be compared by including indirect and direct measures as within-subject factors for a within-subject contrast in the multivariate analysis of covariance. Conflicting results between direct and indirect variables will be discussed.
LIMITATIONS
As long as each device reduces OSA signs and symptoms that are meaningful for the patient, as deemed by the provider and the patient, success would be implied. Researchers will not make that decision but will analyze the end-of-treatment data. Nevertheless, because of the expected sample size, well-supported conclusions based on MAD design will be unlikely. A proof-of-concept initial clinical trial is the first step that could or could not justify similar future efforts but focus on specific MAD designs, titration protocols, and initial mandibular position.
The proposed methodology for this clinical trial is based on the dental practitioner making the decision of who has the proper indications to use a MAD device. To some degree, that is based not only on AHI values but also on symptomatology. The lack of a specific set of more stringent inclusion criteria that could homogenize the sample would reduce the external validity or reflection of what is happening in community dental practices. A proof-of-concept initial clinical trial is the first needed step that could or could not justify similar future efforts but is focused on more stringent case inclusion criteria.
Clinical judgments play into the definition of appliance success, such as whether patient goals were reached (being able to sleep with a bed partner again) or achieving decent AHI reduction in the face of temporomandibular joint symptoms, etc. Such decisions could be perceived as a limitation of this study design. An attempt to control for this is proposed by measuring AHI/ oxygen desaturation index via a level 3 sleep study and categorizing participants accordingly. Objective definitions of success from that data (i.e., 80% reduction in AHI and AHI < 10 or similar) can be applied thereafter.
Pain is a multifaceted experience that multiple causes can trigger. Therefore, it is very tricky to properly measure it. In future studies, a pain index scale could be considered, but such a simplistic approach would not clarify exactly why pain occurs or is triggered.
PUBLIC INVOLVEMENT
The findings of this research are unlikely to be of direct significance to patients using MADs. Although OSA modulates SNA in a manner that may lead to a higher prevalence of cardiovascular disease, heart arrhythmia, and sudden cardiac death, the clinical significance of changes in SNA during OSA interventions is a topic for future prospective research.43 However, the findings of this study may provide researchers and clinicians with more information about how MADs affect the pathophysiology of the nervous system in patients with OSA. Before developing this study protocol, dental professionals and specialists were consulted regarding their clinical practices in delivering MAD therapy, the number of patients they treat with MADs each month, and the perceived clinical benefit of a deeper understanding of the effects of MADs therapy on cardiovascular and neurovascular pathophysiology found in patients with OSA.
It appeared that most clinicians follow a similar clinical protocol and that most of them treat one to two patients using MAD therapy every 1 to 3 months. All clinicians expressed interest in a deeper understanding of the effect of MAD therapy on cardiovascular and neurovascular pathophysiology, which has ultimately driven their willingness to refer patients to this study. Future discussions with dental practitioners will seek feedback on the relevance of findings to their clinical practice and guidance on conducting a randomized controlled trial once initial data are available to direct resources.
ABBREVIATIONS
Apnea-hypopnea index: AHI
Continuous positive airway pressure: CPAP
Electrocardiogram: ECG
Flow-mediated dilation: FMD
Heart rate variability: HRV
Mandibular advancement devices: MAD
Muscle sympathetic nerve activity: MSNA
Nitric oxide: NO
Oxygen desaturation index: ODI
Obstructive sleep apnea: OSA
Parasympathetic nervous system: PNS
Sympathetic nervous activity: SNA
Sympathetic nervous system: SNS
ACKNOWLEDGMENTS
Special thanks are extended to the members of the Neurovascular Health Laboratory at the University of Alberta (A.B., N.C., M.G., B.O., B.M.) for their support in data collection.
REFERENCES
- Peppard PE, Young T, Barnet JH, Palta M, Hagen EW, Hla KM. Increased prevalence of sleep-disordered breathing in adults. Am J Epidemiol.2013;177(9):1006-1014. https://doi.org/10.1093/aje/kws342
- Yeghiazarians Y, Jneid H, Tietjens JR, et al. Obstructive sleep apnea and cardiovascular disease: a scientific statement from the American Heart Association. Circulation. 2021;144(3):e56-e67. https://doi.org/10.1161/CIR.0000000000000988
- Wallin BG, Charkoudian N. Sympathetic neural control of integrated cardiovascular function: Insights from measurement of human sympathetic nerve activity. Muscle Nerve. 2007;36(5):595-614. https://doi.org/10.1002/mus.20831
- Peracaula M, Torres D, Poyatos P, et al. Endothelial dysfunction and cardiovascular risk in obstructive sleep apnea: A review article. Life (Basel). 2022;12(4):537. https://doi.org/10.3390/life12040537
- Dewan NA, Nieto FJ, Somers VK. Intermittent hypoxemia and OSA: Implications for comorbidities. Chest. 2015;147(1):266-274. https://doi.org/10.1378/chest.14-0500
- Roder F, Strotmann J, Fox H, Bitter T, Horstkotte D, Oldenburg O. Interactions of sleep apnea, the autonomic nervous system, and its impact on cardiac arrhythmias. Cur Sleep Med Rep. 2018;4(2):160-169. https://doi.org/10.1007/s40675-018-0117-4
- Drew RC, Sinoway LI. Autonomic control of the heart. In: Robertson D, Biaggioni I, Burnstock G, Low PA, Paton JFR, eds. Primer on the Autonomic Nervous System. 3rd ed. San Diego, CA: Academic Press; 2012:177-180.
- Palma JA, Kaufmann H. Obstructive sleep apnea in adults and the autonomic nervous system. In: Chokroverty S, Cortelli P, eds. Autonomic Nervous System and Sleep. Cham, Germany: Springer; 2021:159-163.
- Lanfranchi P, Somers VK. Obstructive sleep apnea and vascular disease. Respir Res. 2001;2(6):315-319. https://doi.org/10.1186/rr79
- Somers VK, Dyken ME, Clary MP, Abboud FM. Sympathetic neural mechanisms in obstructive sleep apnea. J Clin Invest. 1995;96(4):1897-1904. https://doi.org/10.1172/JCI118235
- Maier LE, Matenchuk BA, Vucenovic A, Sivak A, Davenport MH, Steinback CD. Influence of obstructive sleep apnea severity on muscle sympathetic nerve activity and blood pressure: A systematic review and meta-analysis. Hypertension. 2022;79(9):2091-2104. https://doi.org/10.1161/HYPERTENSIONAHA.122.19288
- Narkiewicz K, Kato M, Phillips BG, Pesek CA, Davison DE, Somers VK. Nocturnal continuous positive airway pressure decreases daytime sympathetic traffic in obstructive sleep apnea. Circulation. 1999;100(23):2332-2335. https://doi.org/10.1161/01.cir.100.23.2332
- Ruzicka M, Knoll G, Leenen FHH, Leech J, Aaron SD, Hiremath S. Effects of CPAP on blood pressure and sympathetic activity in patients with diabetes mellitus, chronic kidney disease, and resistant hypertension. CJC Open. 2020;2(4):258-264. https://doi.org/10.1016/j.cjco.2020.03.010
- Henderson LA, Fatouleh RH, Lundblad LC, McKenzie DK, Macefield VG. Effects of 12 months continuous positive airway pressure on sympathetic activity related brainstem function and structure in obstructive sleep apnea. Front Neurosci. 2016;10:90. https://doi.org/10.3389/fnins.2016.00090
- Rotenberg BW, Murariu D, Pang KP. Trends in CPAP adherence over twenty years of data collection: a flattened curve. J Otolaryngol Head Neck Surg. 2016;45(1):43. https://doi.org/10.1186/s40463-016-0156-0
- De Vries GE, Hoekema A, Houwerzijl E, et al. Cardiovascular effects of a mandibular advancement device versus continuous positive airway pressure in moderate obstructive sleep apnea. Eur Respir J. 2018;52(Suppl62):4344. https://doi.org/10.1183/13993003.congress-2018.PA4344
- Hoekema A, Stegenga B, De Bont LG. Efficacy and co-morbidity of oral appliances in the treatment of obstructive sleep apnea-hypopnea: A systematic review. Crit Rev Oral Biol Med. 2004;15(3):137-155. https://doi.org/10.1177/154411130401500303
- Anandam A, Patil M, Akinnusi M, Jaoude P, El-Solh AA. Cardiovascular mortality in obstructive sleep apnoea treated with continuous positive airway pressure or oral appliance: An observational study. Respirology. 2013;18(8):1184-1190. https://doi.org/10.1111/resp.12140
- Wu H, Zhan X, Zhao M, Wei Y. Mean apnea-hypopnea duration (but not apnea-hypopnea index) is associated with worse hypertension in patients with obstructive sleep apnea. Medicine (Baltimore). 2016;95(48):e5493. https://doi.org/10.1097/MD.0000000000005493
- Punjabi NM, Caffo BS, Goodwin JL, et al. Sleep-disordered breathing and mortality: A prospective cohort study. PLoS Med. 2009;6(8):e100132. https://doi.org/10.1371/journal.pmed.1000132
- Gami AS, Olson EJ, Shen WK, et al. Obstructive sleep apnea and the risk of sudden cardiac death: a longitudinal study of 10,701 adults. J Am Coll Cardiol. 2013;62(7):610-616. https://doi.org/10.1016/j.jacc.2013.04.080
- Marin JM, Carrizo SJ, Vicente E, Agusti AG. Long-term cardiovascular outcomes in men with obstructive sleep apnoea-hypopnoea with or without treatment with continuous positive airway pressure: An observational study. Lancet. 2005;365(9464):1046-1053. https://doi.org/10.1016/S0140-6736(05)71141-7
- Malpas SC. Sympathetic nervous system overactivity and its role in the development of cardiovascular disease. Physiol Rev. 2010;90(2):513-557. https://doi.org/10.1152/physrev.00007.2009
- Heart rate variability: standards of measurement, physiological interpretation and clinical use. Task Force of the European Society of Cardiology and the North American Society of Pacing and Electrophysiology. Circulation. 1996;93(5):1043-1065.
- Meng G, He W, Wong J, et al. Successful continuous positive airway pressure treatment reduces skin sympathetic nerve activity in patients with obstructive sleep apnea. Heart Rhythm. 2022;19(1):127-136. doi: 10.1016/j.hrthm.2021.09.018.
- He W, Tang Y, Meng G, et al. Skin sympathetic nerve activity in patients with obstructive sleep apnea. Heart Rhythm. 2020;17(11):1936-1943. doi: 10.1016/j.hrthm.2020.06.018.
- Grassi G, Esler M. How to assess sympathetic activity in humans. J Hypertens. 1999;17(6):719-734. https://doi.org/10.1097/00004872-199917060-00001
- Mano T. Clinical applications of microneurography. In: Robertson D, Biaggioni I, Burnstock G, Low PA, Paton JFR, eds. Primer on the Autonomic Nervous System. 3rd ed. San Diego, CA: Academic Press; 2012:393-397.
- Zygmunt A, Stanczyk J. Methods of evaluation of autonomic nervous system function. Arch Med Sci. 2010;6(1):11-18. https://doi.org/10.5114/aoms.2010.13500
- Ucak S, Dissanayake HU, Sutherland K, de Chazal P, Cistulli PA. Heart rate variability and obstructive sleep apnea: Current perspectives and novel technologies. J Sleep Res. 2021;30(4):e13274. https://doi.org/10.1111/jsr.13274
- Meah VL, Busch SA, Jones KE, Davenport MH, Steinback CD. A review of acute responses, after-effects and chronic complications related to microneurography. Clin Neurophysiol. 2019;130(10):1781-1788. https://doi.org/10.1016/j.clinph.2019.06.228
- Glos M, Penzel T, Schoebel C, et al. Comparison of effects of OSA treatment by MAD and by CPAP on cardiac autonomic function during daytime. Sleep Breath. 2016;20(2):635-646. https://doi.org/10.1007/s11325-015-1265-0
- Coruzzi P, Gualerzi M, Bernkopf E, et al. Autonomic cardiac modulation in obstructive sleep apnea: effect of an oral jaw-positioning appliance. Chest. 2006;130(5):1362-1368. https://doi.org/10.1378/chest.130.5.1362
- Shiomi T, Guilleminault C, Sasanabe R, Hirota I, Maekawa M, Kobayashi T. Augmented very low frequency component of heart rate variability during obstructive sleep apnea. Sleep. 1996;19(5):370-377. https://doi.org/10.1093/sleep/19.5.370
- Dal-Fabbro C, Garbuio S, D'Almeida V, Cintra FD, Tufik S, Bittencourt L. Mandibular advancement device and CPAP upon cardiovascular parameters in OSA. Sleep Breath. 2014;18(4):749-759. https://doi.org/10.1007/s11325-014-0937-5
- Fukuda T, Tsuiki S, Kobayashi M, Nakayama H, Inoue Y. Selection of response criteria affects the success rate of oral appliance treatment for obstructive sleep apnea. Sleep Med. 2014;15(3):367-370. https://doi.org/10.1016/j.sleep.2013.12.007
- Thijssen DHJ, Bruno RM, van Mil ACCM, et al. Expert consensus and evidence-based recommendations for the assessment of flow-mediated dilation in humans. Eur Heart J. 2019;40(30):2534-2547. https://doi.org/10.1093/eurheartj/ehz350
- Radmand R, Chiang H, Di Giosia M, et al. Defining and measuring compliance with oral appliance therapy. J Dent Sleep Med. 2021;8(3). http://dx.doi.org/10.15331/jdsm.7204
- Fagius J, Wallin BG. Long-term variability and reproducibility of resting human muscle nerve sympathetic activity at rest, as reassessed after a decade. Clin Auton Res. 1993;3(3):201-205. https://doi.org/10.1007/BF01826234
- Flammer AJ, Anderson T, Celermajer DS, et al. The assessment of endothelial function: From research into clinical practice. Circulation. 2012;126(6):753-767. `https://doi.org/10.1161/CIRCULATIONAHA.112.093245
- Wallin BG, Sundlöf G, Eriksson BM, Dominiak P, Grobecker H, Lindblad LE. Plasma noradrenaline correlates to sympathetic muscle nerve activity in normotensive man. Acta Physiol Scand. 1981;111(1):69-73. https://doi.org/10.1111/j.1748-1716.1981.tb06706.x
- Esler M, Jennings G, Korner P, et al. Assessment of human sympathetic nervous system activity from measurements of norepinephrine turnover. Hypertension. 1988;11(1):3-20. https://doi.org/10.1161/01.hyp.11.1.3
- Leung RS. Sleep-disordered breathing: Autonomic mechanisms and arrhythmias. Prog Cardiovasc Dis. 2009;51(4):324-338. https://doi.org/10.1016/j.pcad.2008.06.002
- Task Force of The European Society of Cardiology and The North American Society of Pacing and Electrophysiology . European Heart Journal (1996) 17, 354–381
SUBMISSION & CORRESPONDENCE INFORMATION
Submitted for publication September 15, 2023
Submitted in final revised form January 1, 2024
Accepted for publication April 1, 2024
Address correspondence to: Carlos Flores-Mir, DSc; Professor, Faculty of Medicine and Dentistry, University of Alberta
Address: 5-528 Edmonton Clinic Health Academy
11405 87 Ave, Edmonton, AB T6G 1C9
Canada; Email: cf1@ualberta.ca
DISCLOSURE STATEMENT
These authors contributed equally to this work.
All authors have seen and approved the manuscript. EK conceived and designed the protocol methodology and analysis. SA & CS critically appraised the protocol analysis and provided ongoing laboratory support for data collection. CF critically appraised the protocol methodology.
Emily King was supported by a Motyl Canada Scholarship in Cardiac Sciences, the Fund for Dentistry & McIntyre Fund of the School of Dentistry, and an AADSM Dental Research Award.
No author declares a conflict of interest.
PDF